Biotechnology / Metabolic engineering
Microbiology has always been an applied science, driven by the use of the fascinating biochemical and genetic repertoire of microbes to improve human life sustainably. We use synthetic biology and metabolic engineering to establish our model organisms as production platform for biofuels and other biocommodities and for biological hydrogen storage and conversion. Electrobiotechnology and electrosynthesis catalyzed by acetogenic bacteria is also on our list.
1. Acetogenic bacteria as production platform for biofuels and biocommodities
Acetogenic bacteria grow by the conversion of H2 and CO2 to acetate. Some acetogens, for example Clostridium ljungdahlii, can also produce ethanol. A number of acetogens can also convert synthesis gas (SynGas) (H2 + CO2 + CO) to acetate or ethanol. The capability of acetogens to convert simple gases to ethanol has led to a tremendous interest in using acetogenic bacteria to produce biofuels from H2 + CO2 or SynGas. This process has the advantage that it is not only not depending on renewable carbon sources such as glucose (monomer) or straw (polymer) but that a green house gas is converted to a valuable product. This has led to the 3rd generation in the biotechnology of producing biofuels. Companies such as LanzaTech (www.lanzatech.com) already use this process on an industrial scale. The challenging task for the future is to use the tools of synthetic biology to create strains of acetogens to produce biofuels, such as butanol or biodiesel or other biocommodities such as butanediol or succinat. We are establishing a genetic toolbox to integrate genetic chassis into the genome of A. woodii for the production of the desired products.
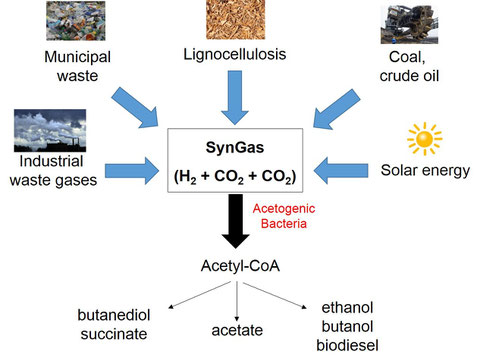
Acetogenic bacteria as production platform for biofuels and biocommodities from SynGas.
References
Poehlein, A., Schmidt, S., Kaster, A.-K., Goenrich, M., Vollmers, J., Thürmer, A., Bertsch, J., Schuchmann, K., Voigt, B., Hecker, M., Daniel, R., Thauer, R.K., Gottschalk, G., Müller, V. (2012) An ancient pathway combining carbon dioxide fixation with the generation and utilization of a sodium ion gradient for ATP synthesis. PLoS ONE 7 : e33439.
2. Biological storage of hydrogen
Storage and transportation of hydrogen is a major obstacle for its use as a fuel. An increasingly considered alternative for the direct handling of hydrogen is to use CO2 as an intermediate storage material. However, CO2 is thermodynamically stable and developed chemical catalysts often require high temperatures, precursors end/or additives for high catalytic rates. We have recently discovered a bacterial hydrogen-dependent CO2 reductase (HDCR) from Acetobacterium woodii that directly catalyzes the hydrogenation of CO2. The HDCR is composed of four subunits harbouring iron sulfur centers as well as a molybdopterin. The enzyme is significantly more effective than the best chemical catalyst. The enzyme also catalyzes the reverse reaction.
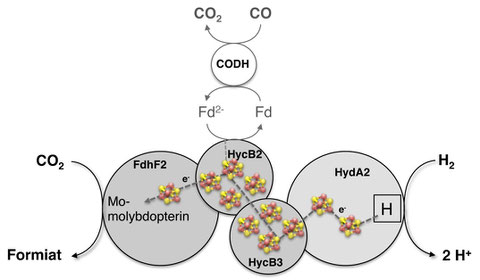
Model of the hydrogen-dependent CO2 reductase of A. woodii. Hydrogen is oxidized by a hyhydrogenase subunit HydA1. The electrons are shuttled to the subunit FdhF2 resopnsible for CO2 reduction. Carbon monoxide can serve as alternative electron donor for CO2 reduction.
We are currently constructing variants of the complex using molecular tools, with the aim to explore and improve yield and stability for applications on a large industrial scale.
We have also engineered A. woodii to produce formate from H2 + CO2 with whole cells. This system is robust and produces formate as sole end product. This opens an avenue for a budget friendly and efficient way of either hydrogen storage and/or formate production.
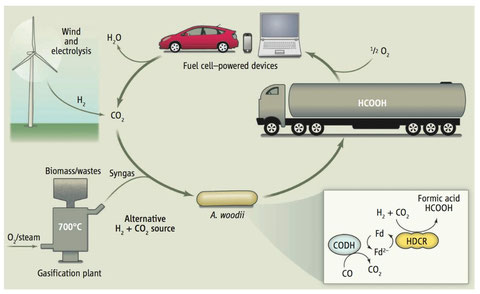
A. woodii as biocatalyst for hydrogen storage. Green hydrogen can be produced with wind energy and electrolysis of water. A. woodii then binds hydrogen to CO2. The product formic acid is liquid at room temperature and can be stored and distributed with existing infrastructure. Eventually, it can then be used as fuel for different applications by release of hydrogen or by direct formic acid fuel cells closing the cycle (Pereira I.A.C., 2013, Science).
References
Schuchmann, K., Müller, V. (2014) Wasserstoff als Energieträger der Zukunft? Bakterien bereiten Weg zu einer biologischen Speicherung des Gases. GIT Labor-Fachzeitschrift 6 : 39-41.
Schuchmann, K., Müller, V. (2014) Ein neues Enzym zur direkten Hydrogenierung von CO2. Biospektrum 20 : 240-241.
Schuchmann, K., Müller, V. (2013) Direct and reversible hydrogenation of CO2 to formate by a bacterial carbon dioxide reductase. Science 342 : 1382-1385.
3. Compatible solutes as additives: Ectoine and hydroxyectoine synthesis
Halophilic organisms are challenged with a potential loss of water: since biological membranes are permeable to water, a higher solute concentration outside a cell will drag water out of the cell, leading to plasmolysis and finally cell death. As a countermeasure and to prevent loss of water, microbes produce compatible solutes, compounds with low molecular mass and high solubility. Typical compatible solutes are glycine betaine, glutamate, proline or ectoine. These compounds not only prevent loss of water from a bacterial cell, but also from eukaryotic cells and ectoine is already used in skin care products as “moisturing” additive.
We have unravelled ectoine biosynthesis in our model organism, Halobacillus halophilus, a moderately halophilic Gram positive bacterium. The genes for ectoine biosynthesis have been identified, the genome sequence is known and a genetic toolbox was established. In the future, we aim to use synthetic biology to establish H. halophilus as an efficient ectoine producer.
Hydroxyectoine is a derivative of ectoine, produced from the latter by action of an ectoin hydroxylase. Hydroxyectoine is superior over ectoine as moisturing agent. H. halophilus has a gene encoding a protein with similarity to ectoine hydroxylases. We overproduced the protein and found that it is indeed an ectoine hydroxylase. The enzyme is currently investigated with a focus on its use in an industrial application.
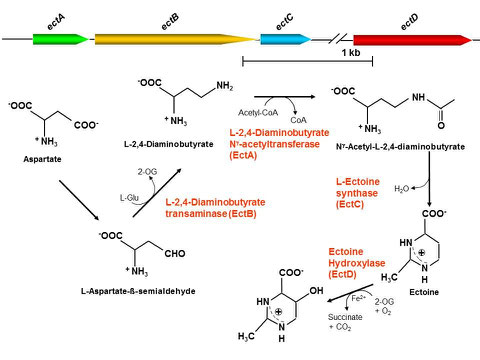
Genetic organization and pathway for the biosynthesis of ectoine and 5-hydroxyectoine in H. halophilus.
References
Saum, S.H., Pfeiffer, F., Palm, P., Rampp, M., Schuster, S.C., Müller, V., Oesterhelt, D. (2012) Chloride and organic osmolytes: a hybrid strategy to cope with elevated salinities by the moderately halophilic, chloride-dependent bacterium Halobacillus halophilus. Environ. Microbiol. 15 : 1619-1633.
Köcher, S., Averhoff, B., Müller, V. (2011) Development of a genetic system for the moderately halophilic bacterium Halobacillus halophilus: Generation and characterization of mutants defect in the production of the compatible solute proline. Environ. Microbiol. 13 : 2122-2131.
Saum, S.H., Müller, V. (2008) Growth phase-dependent switch in osmolyte strategy in a moderate halophile: ectoine is a minor osmolyte but major stationary phase solute in Halobacillus halophilus. Environ. Microbiol. 10 : 716-726.